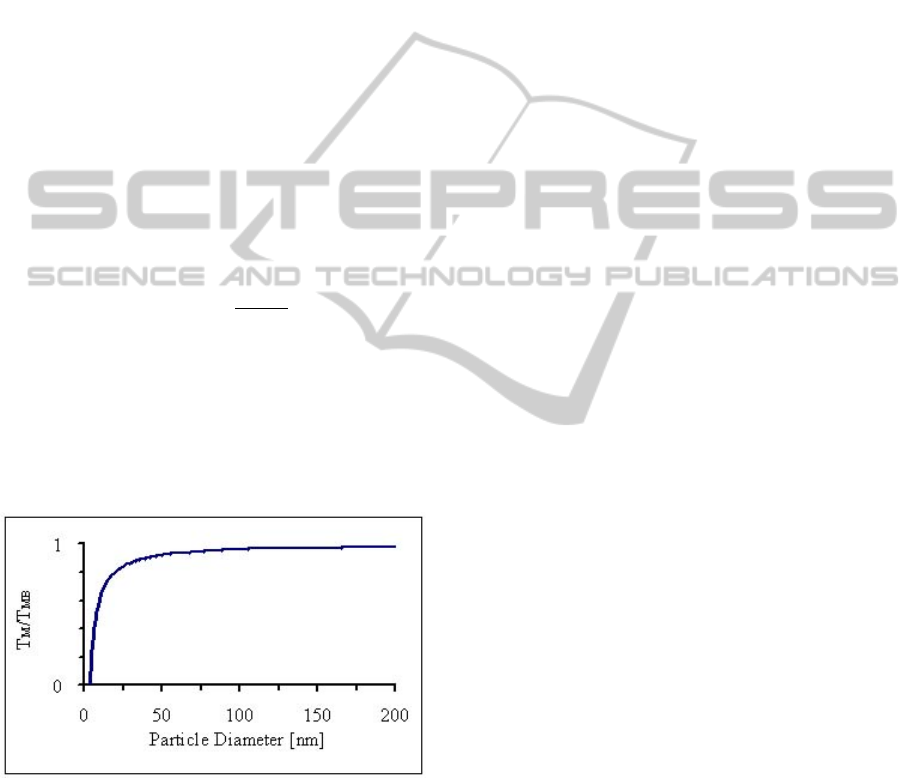
dimensions of the body. As the dimensions of a
material decrease, the melting temperature scales
with the material dimensions (Figure 7).
Nanoparticles have a much greater surface to
volume ratio than bulk materials. The increased
surface to volume ratio means surface atoms have a
much greater effect on chemical and physical
properties of a nanoparticle. Surface atoms bind in
the solid phase with less cohesive energy because
they have fewer neighboring atoms in close
proximity compared to atoms in the bulk of the
solid. Each chemical bond an atom shares with a
neighboring atom provides cohesive energy, so
atoms with fewer bonds and neighboring atoms have
lower cohesive energy. The decrease in melting
temperature can be on the order of tens to hundreds
of degrees compared to the standard melting
temperature of a bulk (T
. The theoretical size-
dependent melting point of a material (T
d
can
be calculated through classical thermodynamic
analysis. The result is the Gibbs-Thomson equation
(Haas, 1936) below:
1
4
(4)
Where σ
is the solid-liquid interface energy, H
is
the bulk heat of fusion, ρ
is density of solid and d is
the particle diameter. Although suspension volume
in this work is larger than nanometer scale, pre-
melting is initiated at the corners and edges of the
crystals (Pan D, 2011), and must be considered in
this work.
Figure 7: A normalized melting curve for gold as a
function of nanoparticle diameter. Experimental melting
curves for near spherical metal nanoparticles exhibit a
similarly shaped curve.
6 EXPECTED OUTCOME
The aim of this work is to understand the unique
physical phenomenon of freezing in very small
volumes, and its effect on lodged live sperm cells.
This knowledge will make it possible to develop a
device, and protocols for freezing and retrieving
small numbers of sperm cells at a pre-selected
location, on a novel cryo-preservation chip. Such an
innovation will improve treatment of male infertility
for those who suffer from Oligo-Terato-
Asthenozoospermia (OTA) syndrome, as well as
those with azoospermia after Testicular Sperm
Extraction (TESE) procedure. Worldwide, there are
about 20,000 IVF labs. At the average, each
laboratory treats about 50 cases of low sperm count
related diseases a year, with unsatisfactory,
unsuitable cryopreservation procedures and means.
In this respects, we strongly believe that overcoming
the above mentioned temporary problems of
cryopreservation of individual sperm cells within
volumes less than nano liter, using our novel device
for cryo-preservation, will ensure there is no loss of
spermatozoa during the freezing-thawing process.
REFERENCES
A. Cesana, P. N. (2003). Sperm cryopreservation in oligo-
asthenospermic patients in Proceedings of
spermatozoa in yolk-filled human zonae pellucidae.
75(no. 4).
A. Herrler, S. E. (2006). Cryopreservation of spermatozoa
in alginic acid capsules. 85(no. 1).
A. Just, I. G. (2004). Novel method for the
cryopreservation of testicular sperm and ejaculated
spermatozoa from patients with severe oligospermia: a
pilot study. Fertility and Sterility, 445-447.
Adamson, M. G. (2001). A method of successful
cryopreservation of small numbers of human
spermatozoa. 76.
C. Quintans, M. D. (2003). Development of a novel
approach for cryopreservation of very small numbers
of spermatozoa. 15.
Cohn, j. R. (2012). Gibbs Thomson Equation . book on
Demand.
D. A. Isaev, S. Y. (2007). Artificial microcontainers for
cryopreservation of solitary spermatozoa.
DeWitt, B. L. (2007). Fundamentals of Heat and Mass
Transfer. John Wiley & Sons.
E. Isachenko, V. I. (2004). DNA integrity and motility of
human spermatozoa after standard slow freezing
versus cryoprotectant-free vitrification. 19(no. 4).
E. Sereni, M. A. (2008). Freezing spermatozoa obtained
by testicular fine needle aspiration: a new technique.
16(no. 1).
F. Nawroth, V. I. (2002). Vitrification of human
spermatozoa without cryoprotectants. 23(no. 2).
Haas, F. G. (1936). A Commentary on the Scientific
Writings of J. Willard Gibbs. New Haven,
Connecticut: Yale University Press, 544.
BIOSTEC2014-DoctoralConsortium
92