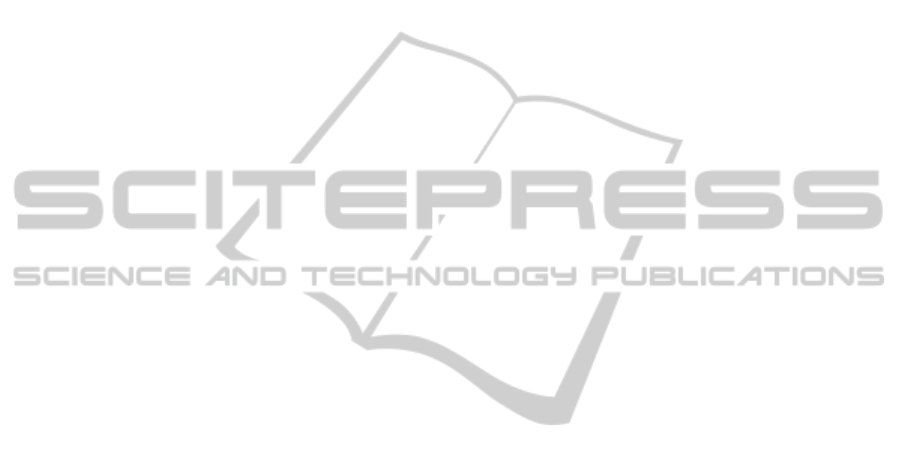
providing physicians with a valuable and non-
invasive assessment tool for bone quality and
strength.
An independent verification of our results is of
course required and foreseen. in this context micro
finite element simulations will be setup, to correlate
our image analysis to compressive strength of the
specimens. a verification of these models will be
based on uniaxial compressive experiments of the
harvested samples, conducted only in the primary
direction (1), due to the destructive nature of the
tests.
Brown et al. (1980) and Martens et al. (1983)
performed compression tests by loading in three
directions: anterior-posterior, superior-inferior and
medial-lateral. They shown that anisotropy was
evident and increase in stiffness was found in the
regions traversed by the primary trabecular system
(Brown et al., 1980); (Martens et al., 1983).
However, in their studies bone specimens were
obtained from nonspecific regions of the entire
femoral heads. in our study we examined eight
different but well defined orientations with similar
positioning within the femoral structure. Sugita et al.
(1999) examined the differences in anisotropy in
osteoporotic bone in the primary compressive group
of the femoral head. They found increased values of
compressive stiffness in the parallel loading group
compared with the perpendicular loading group, but
the anisotropic behaviour of cancellous bone is
reduced, and the femoral head became isotropic as
the bone density decreased (e.g. in osteoporosis).
The anisotropy of vertebral bodies was also
examined in the literature. Mosekilde and Viidik
(1985), found that bone strength was greater in the
vertical than in the transverse direction.
Conclusively, we examined geometrical
anisotropy of trabecular bone and found this to
represent an important characteristic of this severely
inhomogeneous structure. the conversion of our
results with previous experimental findings,
strengthens our hypothesis that micro scale imaging
of the femoral head, at limited spatial resolution,
may be used as an indicator of both, bone strength
and anisotropy.
ACKNOWLEDGEMENTS
The authors would like to thank Dr. A. Tsouknidas
of the Aristotle University of Thessaloniki for his
contribution during the setup and preparation of this
manuscript, as well as Dr. K. Anagnostidis for
providing the bone samples.
REFERENCES
Khosla, S., Riggs, B. L., Atkinson, E. J., Oberg, A.L.,
McDaniel, L. J., Holets, M., Peterson, J. M., Melton,
L.J. 3
rd
, 2006. Effects of sex and age on bone
microstructure at the ultradistal radius: a population-
based noninvasive in vivo assessment. J Bone Miner
Res 21(1), 124-131.
Tsouknidas, A., Michailidis, N., Savvakis, S.,
Anagnostidis, K., Bouzakis, K.-D., Kapetanos, G.,
2012. A finite element model technique to determine
the mechanical response of a lumbar spine segment
under complex loads. J Appl Biomech 28 (4), 448-
456.
Rockwood, P. R., Horne, J. G., Cryer, C., 1990. Hip
Fractures: a future epidemic? J Orthop Trauma 4, 388-
396.
Cooper, C., Campion, G., Melton, L. J., 1992. Hip
fractures in the elderly: a word- wide projection.
Osteopor Int 2, 285-289.
Lotz, J. C., Cheal, E. J., 1995. Hayes W. C. Stress
distribution within the proximal femur during gait and
falls: implication for osteoporotic fracture. Osteopor
Int 5, 252-261.
Raisz, L., 2005. Pathogenesis of osteoporosis: concepts,
conflicts, and prospects. Journal of Clinical
Investigation 115 (12), 3318–3325.
Tsouknidas, A., Anagnostidis, K., Maliaris, G.,
Michailidis, N., 2012. Fracture risk in the femoral hip
region: a finite element analysis supported
experimental approach. J Biomech 45 (11), 1959-
1964.
Tsouknidas, A., Maropoulos, S., Savvakis, S., Michailidis,
N., 2011. FEM assisted evaluation of PMMA and
Ti6Al4V as materials for cranioplasty resulting
mechanical behaviour and the neurocranial protection.
Bio-Med Mater Eng 21 (3), 139-147.
Nauman, E. A., Fong, K. E., Keaveny, T. M., 1999.
Dependence of intertrabecular permeability on flow
direction and anatomic site. Ann Biomed Eng. 27(4),
517-524.
Bevill, G., Keaveney, T. M., 2009. Trabecular bone
strength predictions using finite element analysis of
micro-scale images at limited spatial resolution. Bone
44, 579-584.
Rathnayaka. K., Sahama, T., Schuetz, M. A., Schmutz, B.,
2010. Effects of CT image segmentation methods on
the accuracy of long bone 3D reconstructions. Med
Eng Phys 33(2), 226–233.
Canny, J., 1986. A computational approach to edge
detection. IEEE T Pattern Anal 8(6), 679–698.
Baroud, G., Falk, R., Crookshank, M., Sponagel, S.,
Steffen, T., 2004. Experimental and theoretical
investigation of directional permeability of human
vertebral cancellous bone for cement infiltration, J
Biomech 37(2), 189-196.
Thomas, D. B., Daniel, T. S., 1983. In vitro contact stress
distributions in the natural human hip. J Biomech 16,
373-384.
Hodge, W. H., Fijan, R. S., Carlson, K. L., Burgess, R. G.,
BIOINFORMATICS2013-InternationalConferenceonBioinformaticsModels,MethodsandAlgorithms
240