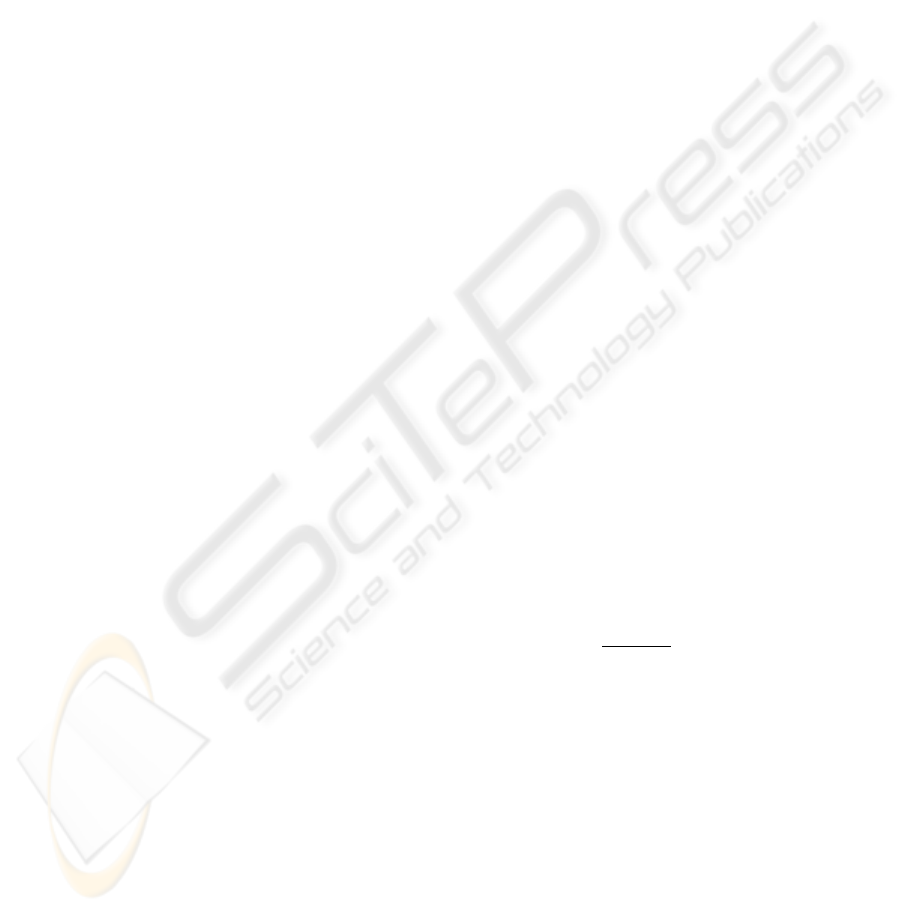
Chapuis, B., Vidal-Petio, E., Orea, V., Barres, C., &
Julien, C., 2004, Linear modelling analysis of
baroreflex control of arterial pressure variability in
rats, J Physiol 559: 639–649.
Chernigovskaya, N. V., Vaschillo, E. G., Rusanovsky, V.
V., & Kashkarova, O. E., 1990, Instrumental
autotraining of mechanisms for cardiovascular
function regulation in treatment of neurotics, The SS
Korsakov's Journal of Neuropathology and Psychiatry,
90: 24–28.
Clynes, M., 1960, Respiratory sinus arrhythmia: laws
derived from computer simulation, Journal of Applied
Physiology,15(5): 863-874.
Codispoti, M., Bradley, M. M., & Lang, P., 2001, Affective
reactions to briefly presented pictures,
Psychophysiology, 38, 474-678.
Dworkin, B. R., Elbert, T., Rau, H., Birbaumer, N., Pauli,
P., Droste, C., & Brunia, C. H., 1994, Central effects
of baroreceptor activation in humans: attenuation of
skeletal reflexes and pain perception, Proc Natl Acad
Sci U S A, 91, 6329-33.
France, C. R., France, J. L., & Patterson, S. M., 2006,
Blood pressure and cerebral oxygenation responses to
skeletal muscle tension: a comparison of two physical
maneuvers to prevent vasovagal reactions, Clinical
Physiology and Functional Imaging, 26, 21–25.
Hassett, A. L, Radvanski, D. C., Vaschillo, E., Vaschillo,
B., Sigal, L., Karavidas, M., Buyske, S., & Lehrer, P.
M., 2007, A pilot study of the efficacy of heart rate
variability biofeedback in patients with fibromyalgia
syndrome, Appl Psychophysiol Biofeedback, 32, 1-10.
Jones, P. P., Christou, D. D., Jordan, J., & Seals, D. R.,
2003, Baroreflex buffering is reduced with age in
healthy men, Circulation, 107, 1770–1774.
Jordan, J., Tank. J., Shannon, J. R., Diedrich, A., Lipp, A.,
Schroder, C., Arnold, G., Sharma, A. M., Biaggioni, I.,
Robertson, D., & Luft, F. C., 2002, Baroreflex
buffering and susceptibility to vasoactive drugs,
Circulation, 105, 1459–1464.
Julien, C., 2006, The enigma of Mayer waves: Facts and
models, Cardiovasc Res, 70, 12–21.
Just, A., Wittmann, U., Nafz, B., Wagner, C. D., Ehmke,
H., Kirchheim, H. R, & Persson, P. B., 1994, The
blood pressure buffering capacity of nitric oxide by
comparison to the baroreceptor reflex, Am J Physiol
Heart Circ Physiol 267: H521–H527.
Karavidas, M. K., Lehrer, P. M., Vaschillo, E., Vaschillo,
B., Marin, H., Buyske, S., Radvanski, D., & Hasset,
A., 2007, Preliminary results of an open label study of
heart rate variability for the treatment of major
depression, Appl Psychophysiol Biofeedback, 32, 19-
30.
Lang, P. J., Greenwald, M. K., & Bradley, M. M., 1993.
Looking at pictures: Affective, facial, visceral, and
behavioral reactions, Psychophysiology, 30, 261-273.
Lehrer, P. M., Vaschillo, E., & Vaschillo, B., 2000,
Resonant Frequency Biofeedback Training to Increase
Cardiac Variability: Rationale and Manual for
Training, Appl Psychophysiol Biofeedback, 25, 181-
192.
Lehrer, P. M., Vaschillo, E., Vaschillo, B., Lu, S. E.,
Eckberg, D. L., Edelberg, R., Shih, W. J., Lin, Y.,
Kuusela, T. A., Tahvanainen, K. U. O., & Hamer, R.,
2003, Heart rate variability biofeedback increases
baroreflex gain and peak expiratory flow,
Psychosomatic Medicine, 65, 796–805.
Lehrer, P., Vaschillo, E., Vaschillo, B., Lu, S., Scardella,
A., Siddique, M., & Habib, R.., 2004, Biofeedback
treatment for asthma, Chest, 126, 352–361.
Magosso, E., Biavati, V., & Ursino, N., 2001, Analysis of
cardiovascular instability by mathematical model of
baroreflex control, Proceedings of the 23
rd
Annual
EMBS International Conference, October 25-28,
Istanbul, Turkey. 596-599.
McCraty, R., Atkinson, M., & Tomasino, D., 2003, Impact
of a workplace stress reduction program on blood
pressure and emotional health in hypertensive
employees, J Altern Complement Med, 9, 355–369.
Nyklicek, I., Wijnen, V., & Rau, H., 2005, Effects of
baroreceptor stimulation and opioids on the auditory
startle reflex, Psychophysiology, 42, 213-22.
van de Vooren, H., Gademan, M. G. J., Swenne, C. A.,
TenVoorde, B. J., Schalij, N. J., & Van der Wall, E.
E., 2007, Baroreflex sensitivity, blood pressure
buffering, and resonance: what are the links?
Computer simulation of healthy subjects and heart
failure patients, J Appl Physiol 102: 1348-1356.
Vaschillo, E. G., Bates, M. E., Vaschillo, B., Lehrer, P.,
Udo, T., Mun, E. Y., & Ray, S., 2008, Heart rate
variability response to alcohol, placebo, and
emotional picture cue challenges: Effects of 0.1 Hz
stimulation, Psychophysiology, 45, 847-858.
Vaschillo, E., Vaschillo, B., & Lehrer, P., 2006,
Characteristics of resonance in heart rate variability
stimulated by biofeedback, Appl Psychophysiol
Biofeedback, 31, 129-142.
Vaschillo, E., Lehrer, P., Rishe, N., & Konstantinov, M.,
2002, Heart rate variability biofeedback as a method
for assessing baroreflex function: a preliminary study
of resonance in the cardiovascular system, Appl
Psychophysiol Biofeedback, 27, 1–27.
Vashchillo, E. G., Zingerman, A. M., Konstantinov, M.
A., & Menitsky, D. N., 1983, An investigation of the
resonance characteristics of the cardiovascular
system, Human Physiology, 9, 257-265.
Vaschillo, E. G. & Vaschillo
, B., 2009, Transfer function
of the heart rate control system with respiratory input.
The classical engineering approach, BIOSIGNALS
2009, 233-238.
Ursino, M. & Magosso, E., 2003, Role of short-term
cardiovascular regulation in heart period variability:
a modelling study, Am J Physiol – Heart and
Circulatory Physiology, 284, H1479-H1493.
Yasumasu, T., Reyes del Paso, G., Takahara, K., &
Nakashima, Y., 2006, Reduced baroreflex cardiac
sensitivity predicts increased cognitive performance,
Psychophysiology 43: 41-45.
BIOSIGNALS 2010 - International Conference on Bio-inspired Systems and Signal Processing
28