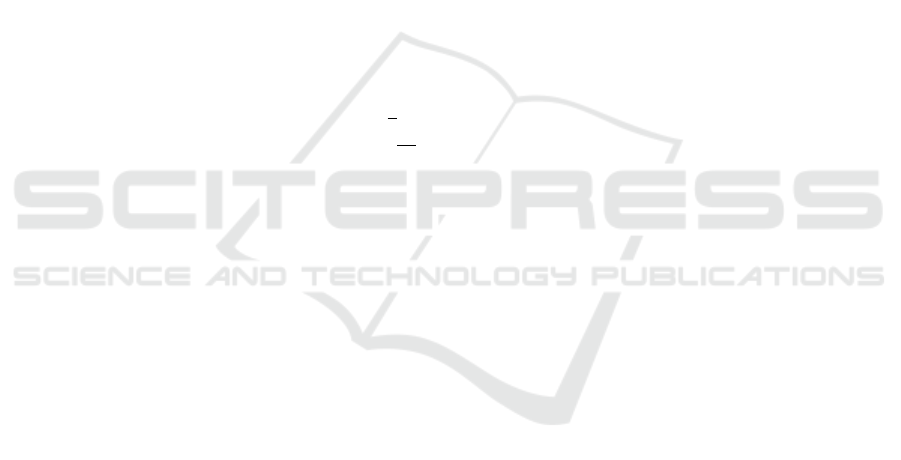
One solution would be to use VCSELs with a fre-
quency split so low that it cannot be resolved within
experimental accuracy. Another way to counter the
spectral attack is to use two identical VCSELs with
orthogonally polarized modes having the same fre-
quencies
f
LOW
and
f
HIGH
. This means that there is
no one-to-one correspondence between frequency and
polarization – in fact, they are independent. There-
fore Eve is unable to gain information by performing
a spectral measurement on the qubits. She can learn
which frequency is assigned to which bit value in a cer-
tain basis after Alice and Bob publicly disclose their
basis choices, but unless she finds a way to measure
a photon’s frequency without destroying it, the eaves-
dropping would be ineffective without a polarization
measurement, which can be revealed by the protocol.
To offer protection even against non-destructive
frequency measurement attacks, different bit values
can be assigned to the same (low or high) frequency
mode (Table 2) in case of the two lasers, so that the
bit value and the frequency are independent as well.
Note that non-destructive frequency measurements
still change the photon’s wavefunction according to
the time-energy uncertainty relation
∆E · ∆t ≥
~
2
. If
the measurement has high precision (small
∆ω =
∆E
~
),
∆t
becomes large. This means further problems for the
eavesdropper, because it increases the probability of
detecting the photon in a wrong time bin, thereby the
error rate.
5 CONCLUSION
Vertical cavity surface-emitting lasers have several ad-
vantages over traditional edge-emitting lasers in a low-
power quantum key distribution scheme. However,
their inherent polarization switching mechanism may
cause problems as several protocols use polarization
qubits. This requires the ability to output photons with
controlled polarization. Over the past decades, several
different methods were developed to fix VCSELs’ po-
larization, out of which surface gratings has proven
to provide the best solution without sacrificing the
aforementioned beneficial properties.
Polarization switching, which occurs between two
orthogonal linear polarizations, could also be poten-
tially exploited to simplify QKD transmitters. In this
paper, we proposed a new transmitter design for the
BB84 protocol including only two VCSELs (as op-
posed to four lasers in a trivial design) driven by dif-
ferent amplitude current pulses in order to select the
desired polarization for the output light. Compared to
the trivial BB84 design, this scheme comes with an
extra concern. As the differently polarized modes have
different frequencies, eavesdroppers may perform a
spectral attack. This attack can be negated by using
two identical VCSELs, removing the one-to-one cor-
respondence between frequency and polarization. The
validity of this proposal is still subject to experimental
investigation, the main concern being the maximal key
rate that can be achieved by this transmitter.
ACKNOWLEDGEMENTS
The authors would like to thank Dr. Zsolt Kis for the
helpful suggestions regarding the physics and back-
ground of photon frequency measurements.
This research was supported by the National Re-
search Development and Innovation Office of Hungary
within the Quantum Technology National Excellence
Program (Project No. 2017-1.2.1-NKP-2017-00001).
REFERENCES
Bandyopadhyay, S., Hong, Y., Spencer, P., and Shore, K.
(2003). Vcsel polarization control by optical injection.
Journal of lightwave technology, 21(10):2395–2404.
Barve, A. V., Mehta, A., Husain, A., and Coldren, L. (2014).
Ultrafast electrical polarization modulation in vcsel
with asymmetric current injection. In Optical Intercon-
nects Conference, 2014 IEEE, pages 91–92. IEEE.
Bennett, C. H. (1992). Quantum cryptography using any
two nonorthogonal states. Physical review letters,
68(21):3121.
Bennett, C. H., Bessette, F., Brassard, G., Salvail, L., and
Smolin, J. (1992). Experimental quantum cryptogra-
phy. Journal of cryptology, 5(1):3–28.
Bennett, C. H. and Brassard, G. (1984). Quantum cryptogra-
phy: Public key distribution and coin tossing. In Pro-
ceedings of IEEE International Conference on Com-
puters, Systems and Signal Processing, volume 175,
page 8. IEEE.
Brassard, G., Lütkenhaus, N., Mor, T., and Sanders, B. C.
(2000). Limitations on practical quantum cryptography.
Physical Review Letters, 85(6):1330.
Choquette, K. D., Lear, K., Leibenguth, R., and Asom,
M. (1994). Polarization modulation of cruciform
vertical-cavity laser diodes. Applied physics letters,
64(21):2767–2769.
Choquette, K. D., Schneider, R. P., Lear, K. L., and
Leibenguth, R. E. (1995). Gain-dependent polarization
properties of vertical-cavity lasers. IEEE Journal of
Selected Topics in Quantum Electronics, 1(2):661–666.
Ekert, A. K. (1991). Quantum cryptography based on Bell’s
theorem. Physical review letters, 67(6):661.
Glauber, R. J. (1963). Coherent and incoherent states of the
radiation field. Physical Review, 131(6):2766.
Transmitter Design Proposal for the BB84 Quantum Key Distribution Protocol using Polarization Modulated Vertical Cavity
Surface-emitting Lasers
257