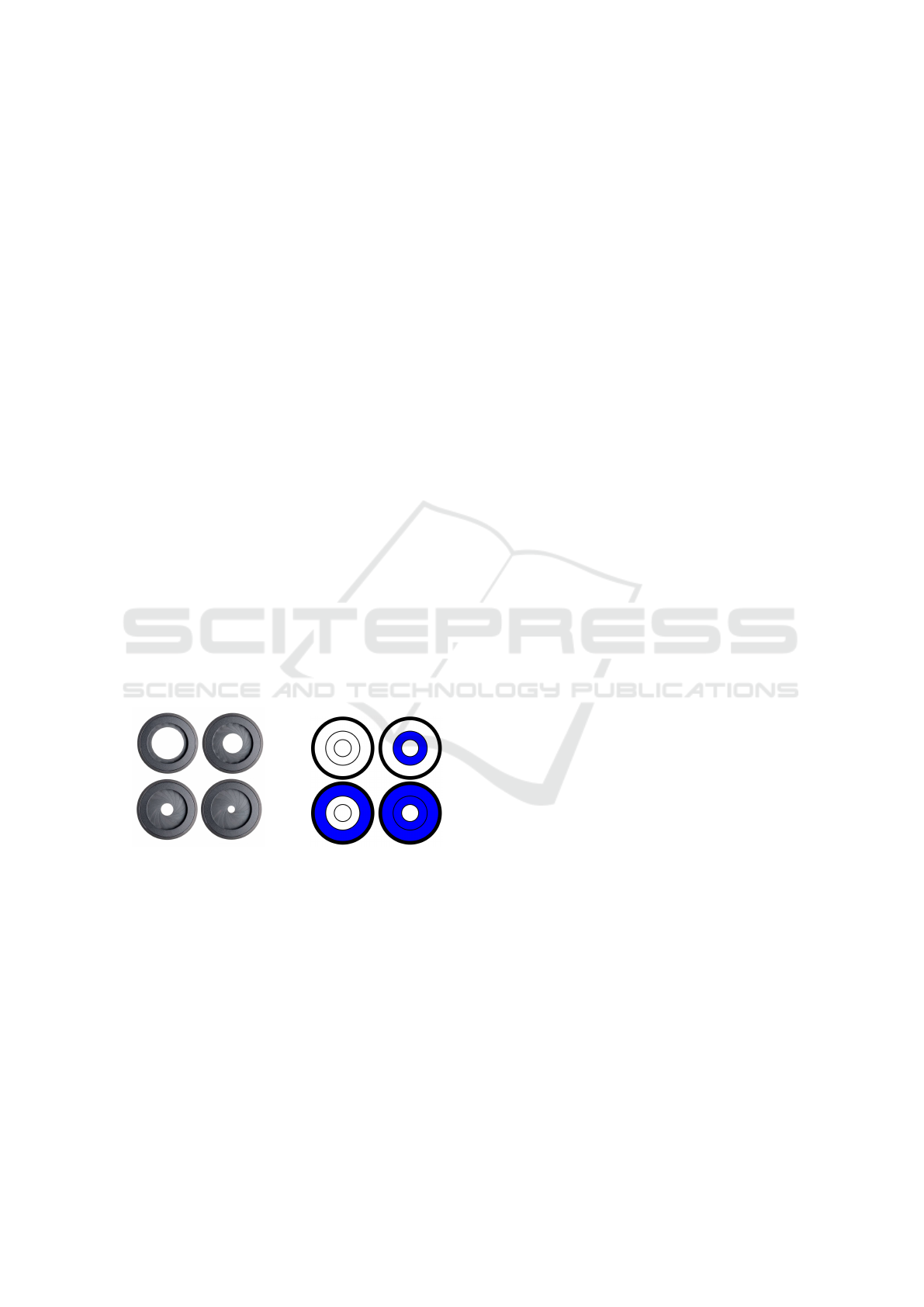
Titanium Dioxide based Electrochromic Iris
Preparation, Characterization and Application
C. Kortz, A. Hein and E. Oesterschulze
Physics and Technology of Nanostructures, University of Kaiserslautern, Erwin-Schroedinger-Strasse 46,
67663 Kaiserslautern, Germany
Keywords:
Miniaturized Iris, Electrochromism, Viologen, Titanium Dioxide.
Abstract:
The miniaturization of a classical iris consisting of blades which are moved towards the path of light is limited,
due to the size of the actuators and the additional space needed for blade storage when the iris is completely
open. To overcome these limitations we present a fast switching, non-mechanical micro iris based on elec-
trochromic molecules, namely viologens, which are adsorbed onto a titanium dioxide nanoporous electrode.
Measurements of the energy consumption, the response time and the spectral light transmission are presented.
The complete fabrication route and the life time of the device are discussed in detail.
1 INTRODUCTION
The realization of a miniaturized iris comes to interest
since more and more commercially available hand-
held electronics were equipped with integrated cam-
eras. Due to the small size of these cameras a classical
blade iris cannot be integrated. An active iris is indis-
pensable for both the intensity and in particular the
depth of focus control.
a) b)
Figure 1: a) Images of a classical iris. In the upper left
corner the iris is completely open and only the blade storage
is left to be seen. This extra space is too big for micro iris
applications for integrated camera systems. b) A schematic
view of an electrochromic iris: the device can be switched
completely transparent, only a small area around the iris is
needed for sealing. This device can be miniaturized to fit
the conditions in integrated optics.
The major reason why there is no iris integrated
in small cameras is the required space. Especially
when the iris is completely open the blades have to be
moved outside the path of light and have to be stored
in an additional ring shaped space (see Fig. 1a)),
which is not available in micro optical devices.
Different solutions were presented to build up
miniaturised iris devices based on microelectrome-
chanical systems (MEMS), liquid displacement tech-
niques and electrochromic devices (Syms et al., 2004)
(Kimmle et al., 2011) (Deutschmann et al., 2015).
MEMS based iris devices have a similar working
principle as a classical blade iris (Yu et al., 2012). A
shutter is moved towards the path of light to realize
the iris function. The advantage of MEMS based de-
vices is the fast switching time. They can be miniatur-
ized easily but the dimensions of the device are much
larger than the path of light and high voltages of more
than 80 V are needed to move the blades. So they do
not fit the conditions for integrated optics in battery
powered hand-held electronics.
Another approach is to move light absorbing liq-
uids, like oil or ink into the path of light to create an
iris (Kimmle et al., 2011). The advantage is that in
this case the storage does not have to be ring shaped
around the optical path, but can be designed freely.
Nevertheless an additional space and actuators have
to be provided (M
¨
uller et al., 2012).
2 STATE OF THE ART
One approach to create a non-mechanical iris was us-
ing electrochromic molecules which change their ab-
sorption by applying a chemical potential (Roth et al.,
2011). Multiple designs and molecules were tested
(Deutschmann and Oesterschulze, 2014). The latest
iris was based on two complementary electrochromic
molecules, which were dissolved in the electrolyte en-
capsulated between two transparent conducting ox-
50
Kortz, C., Hein, A. and Oesterschulze, E.
Titanium Dioxide based Electrochromic Iris - Preparation, Characterization and Application.
DOI: 10.5220/0006552200500054
In Proceedings of the 6th International Conference on Photonics, Optics and Laser Technology (PHOTOPTICS 2018), pages 50-54
ISBN: 978-989-758-286-8
Copyright © 2018 by SCITEPRESS – Science and Technology Publications, Lda. All rights reserved