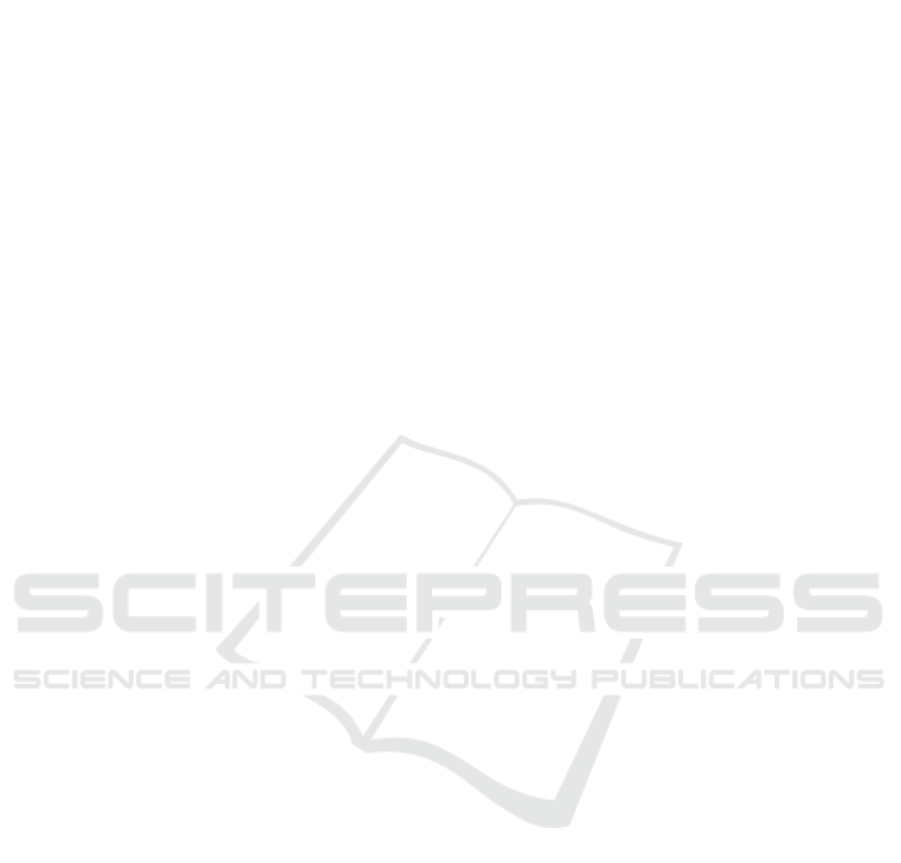
subsurface band bending, drift and diffusion currents
have different signs. At the dye – silicon
heterojunction the change of photopotential character
during illumination in different spectral regions was
discovered (Komolov, et al, 2006). The subsurface
band bending in semiconductors can be controlled by
an external electric field or an additional illumination
(Akimov, 1966), as well as the adsorption of the
electron-acceptor and electron-donor molecules on
the semiconductor surface (Pimenov and Goryaev,
1976).
4 CONCLUSIONS
Observed effective dye sensitization of the internal
photoelectric effect in silicon can be used in such
semiconductor devices for converting the light energy
into electric energy as solar panels or image sensors
in modern electronic photography systems. In the
case of silicon solar batteries increasing the near-
surface free carriers generation may decrease the
thickness of cells. The application of dye sensitization
in CCD matrices will enable the improvement of the
color separation system during the recording of
colored images as in traditional silver halide
photography. Selecting appropriate dyes to provide
an effective increase in sensitivity in the required
spectral range instead of using light filters can
decrease losses of energy and of valuable information
in electronic photography.
ACKNOWLEDGEMENTS
This research was supported by the Ministry of
Education and Science of the Russian Federation
(Project No. 3.5005.2017/BY).
REFERENCES
Afanasyev, V.P., Terukov, E.I., Sherchenkov, A.A., 2011.
Thin Film Solar Cells Based on Silicon. State
Electrotech. Univ. St. Petersburg.
Akimov, I.A., 1966. The internal photoeffect investigation
in semiconductors by the condenser method. In Soviet
J. Optical Technology. V. 33. No 5. P. 248-257.
Akimov, I.A., Cherkasov, Yu.A., Cherkashin, M.I., 1980.
Sensitized Photoeffect. Nauka. Moscow.
Akimov, I.A., Goryaev, М.А., 1984. Photoprocesses in
semiconductors with adsorbed dye. In Zh. fizicheskoi
khimii, V. 58, No 5. P.1104-1107.
Alferov, Zh.I., Andreev, V.M., Rumyantsev, V.D., 2004.
Solar Photovoltaics: Trends and Prospects. In
Semiconductors. V. 38. No 8. P. 899-908.
Boyle, W.S., 2010. Nobel Lecture: CCD—An extension of
man’s view. In Rev. Modern Physics. V. 82, fasc. 3. P.
2305—2306.
Duffie, J.A., Beckman, W.A., 2013. Solar Engineering of
Thermal Processes, John Wiley & Sons, Inc. New
York.
Ermolaev, V.L., Bodunov, E.N., Sveshnikova, E.B. 1996.
Inductive-resonant mechanism of nonradiative transi-
tions in ions and molecules in condensed phase. In
Physics-Uspehi, V. 39. No 3. P. 261-282.
Goryaev, М., 2013. Physical fundamentals of solid state
photochemistry. Photolysis of inorganic solids.
Lambert Acad. Publ., Saarbrucken.
Goryaev, М.А., Dudnikov, Yu.A., 1990. Electronic and
hybrid imaging systems. In Zh. nauchnoi i prikladnoi
fotografii i kinematografii. V. 35. No 5. P. 386-394.
Goryaev, М.А., 2015. Spectral Sensitization of Internal
Photoeffect in Silicon. In Izvestiya Rossiiskogo
gosudarstvennogo pedagogicheskogo universiteta. V.
176. P. 71-75.
Goryaev, М.А., 2015. Dye sensitization of
photoconductivity of polycrystalline silicon. In Russian
J. Physical Chemistry A. V. 89. No. 12. P. 2320-2321.
Goryaev, М.А., 2017. Dye Sensitization of Silicon. In
International J. Modern Engineering Research. V. 7.
No 5. P. 39-43.
Goryaev, М.А., 1980. Method of determining the
luminescence spectra of powdered systems. In Soviet
Technical Physics Letters, V. 6. No 9. P. 484-485.
Goryaev, М.А., 1981. Quantum yield and luminescence
spectra of dyes in the adsorbed state. In Optics and
Spectroscopy. V. 51. No 6. P. 562-564.
Goryaev, М.А., 1997. Spectral dependence of the
luminescence quantum yield of adsorbed dyes. In
Optics and Spectroscopy. V. 82. No 5. P. 723-725.
Goryaev, М.А., Smirnov, A.P., 2015. Luminescence of dye
adsorbed on silver stearate and sensitization of
photothermographic materials. In Izvestiya Rossiiskogo
gosudarstvennogo pedagogicheskogo universiteta. V.
173. P. 50-54.
Goryaev, М.А., Pimenov, Yu.D., 1975. The action of an
electric field on photochemical processes in aluminum
hydride. In Soviet J. Optical Technology. V. 42. No 9.
P. 538-541.
Goryaev, М.А., 1980. Photoprocesses in aluminum hydride
with an adsorbed dye. In Optics and Spectroscopy. V.
49. No 6. P. 625-627.
Goryaev, М.А., 2000. Vacancy mechanism of the
photolysis of aluminum hydride. In Optics and
Spectroscopy. V. 88. No 1. P. 42-45.
Gratzel, M., 2003. Dye-sensitized Solar Cells. In J.
Photochem. Photobiol. C: Photochem. Rev. V. 4. No 2.
P. 145-153.
James, T.H., 1977. The Theory of the Photographic
Process, Мacmillan Publ. Company. New York.
Komolov, S.A., Gerasimova, N.B., Aliev Yu.G., et al,
2006. Photoelectronic properties of organic films on the
PHOTOPTICS 2018 - 6th International Conference on Photonics, Optics and Laser Technology
134