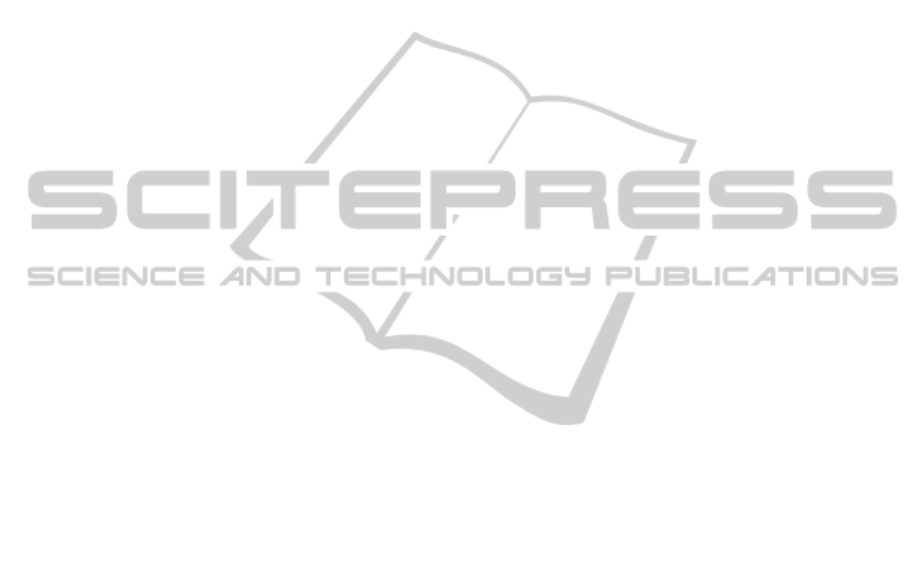
2.2 Instruments and Materials
Treadmill tests were performed on a 5x3 m motor
driven treadmill (Forcelink B.V., Culemborg, the
Netherlands). All skiers used a modified Madshus
Nano Carbon skate boot (Madshus/K2, Biri,
Norways) that was fit to a prototype binding
designed specifically for this project, (IDT Sports,
Lena, Norway). All skiers used the same pair of
roller skis (IDT Skate, IDT Sports, Lena, Norway).
Ventilatory variables were assessed employing
open-circuit indirect calorimetry with an Oxycon
Pro apparatus (Jaeger GmbH, Hoechberg,
Germany). The instruments were calibrated against
ambient air and commercial gas with known
concentrations (16.00 ± 0.04% O
2
and 5.00 ± 0.1%
CO
2
, Riessner-Gase GmbH and Co, Lichtenfels,
Germany).
Movement kinematics were monitored via an
Oqus (Qualisys AB, Gothenburg, Sweden) infrared
camera-system with a 250Hz sample rate and where
synchronized with EMG, sampled at 1500Hz. EMG-
electrodes were attached to the skiers’ dominant
skiing-side leg on the bellies of m. semitendenosus,
m. vastus medialis, m. vastus lateralis, m. rectus
femoris, m. lateral gastrocnemius, and m. tibialis
anterior. The placement of the electrodes followed
the recommendations of the Surface
Electromyography for the Non-Invasive Assessment
of Muscles (SENIAM). The electrodes were bipolar,
disposable pre-gelled Ag/AgCl surface electrodes
(Noraxon USA, Inc., Scottsdale, AZ) with 20 mm
inter-electrode distance.
2.3 Protocols and Procedures
Participants were prepped with retrorflective
markers along with EMG electrodes and sensors
prior to a 20-minute ski specific warm-up with the
normal roller ski boots and bindings on the roller-ski
treadmill. Participants performed three tests utilizing
varying hinge positions: front (toe attachment 0 cm),
middle (4 cm behind toe), back (8 cm behind toe).
Skiers performed 4 min at moderate intensity in G3
skating technique with all hinge positions. All tests
were performed at 5% incline and 3.89 m/s.
Ventilatory variables where continuously monitored
through the entire test. A 15-minute break was given
between hinge tests to minimize sweating and
fatigue.
A skate cycle was defined as one right and one
left leg push-off. The beginning and end of each
cycle was defined as ski lift-off of the left ski. Cycle
rate was calculated as the number of cycles per
second. Cycle length was the covered distance on
the treadmill during one cycle, which was calculated
as speed times cycle time.
Work rate was calculated, in accordance with
Sandbakk et al. (Asan Grasaas et al., 2014),
as the
sum of power against gravity P
g = m ·g · sin α · v
and friction Pf = m · g · cos α · μ · v where m is the
body mass of the skier, g the gravitational
acceleration, α the angle of treadmill incline, v the
speed of the treadmill belt, μ the frictional
coefficient (.026). The aerobic metabolic rate was
calculated as the product of VO
2 and the oxygen
energetic equivalent using the associated respiratory
exchange ratio and standard conversion tables.
Gross
efficiency was calculated as the external work rate
performed by the entire body divided by the aerobic
metabolic rate, presented as a percentage.
The EMG analysis was conducted with custom-
written Matlab
TM
(The MathWorks Inc., Natwick,
MA) codes and included the following steps: For
each trial, 30 stride cycles were determined using the
acceleration signal with the ski takeoff serving as
trigger points. A wavelet transformation (von
Tscharner, 2000) yielded the intensity of the EMG
signal. This intensity was resampled such that 501
data points represented each cycle and normalized to
unit intensity per cycle. The normalized waveforms
of the 6 muscles were then concatenated into a 3506-
dimensional vector. All vectors from the 3 subjects
skating with 3 different hinge positions formed a
270 x 3501 matrix which was submitted to a
principal component analysis (PCA). The PCA
yielded (a) eigenvectors v
k
that quantified correlated
deviations from the mean waveform, (b) eigenvalues
EV
k
quantifying how much of the variability was
represented by each eigenvector, (c) scores ξ
k,i
that
quantified how much the deviations of each cycle i
from the mean waveform was represented by the
eigenvectors v
k
. The scores ξ
k,i
were analyzed to
determine if the hinge position caused correlated
deviations from the mean multi-muscle EMG
waveform. Due to the small number of participants,
the statistical analysis was only conducted to
determine intra-subject effects of the hinge position,
i.e. for each subject a 1-way ANOVA was calculated
and Student T-tests were used for the post-hoc
comparison between hinge positions.
3 RESULTS
The mean cycle lengths for the front, middle and
back hinge positions were 8.0 m, 8.0 m, and 7.8 m
respectively, with corresponding cycle rates of