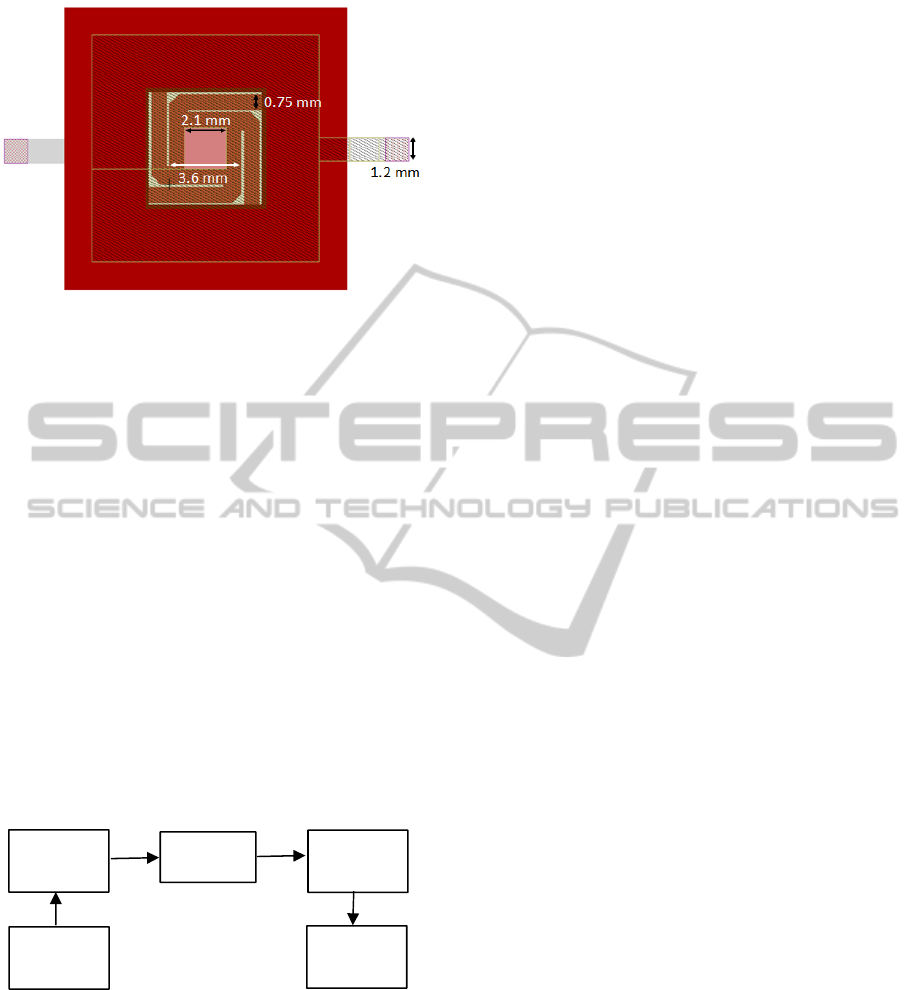
Figure 10: The layout of the tunable FP filter.
2.5 Testing System
In order to control the voltage between the top and
bottom capacitor planes, a Korad voltage supply
KA6003P can be used as reference. The voltage
supply is a programmable single-channel, constant-
voltage power supply with low noise, high reliability
and high accuracy.
The glucose sensor system consists of a voltage
supply, tunable FP filter, detector, testing circuit,
and PC as shown in Fig. 11. The reference detector
is a thermopile detector MLX90614 from Melexis.
The MLX90614 is an Infrared thermometer for non-
contact temperature measurements. Both the IR
sensitive thermopile detector chip and the signal
conditioning ASIC are integrated in the same TO-39
can.
Evaluation board EVB90614 from Melexis is
used as the testing circuit which includes an easy
interface between the MLX90614 infrared
thermometer in TO-can and a PC.
Figure 11: The schematic of glucose sensor system.
3 CONCLUSIONS
In summary, a tunable MEMS Fabry-Pérot filter for
the Long-Wave Infrared range is proposed and
simulated. By applying a voltage ranging from 0 V
to 20 V, the tuning range of output transmission
peak shift from 10.9 µm to 12.19 µm is obtained.
The tunable FP filter is the key part of glucose
sensor and has other applications in hyperspectral
imaging, spectrometers, telecommunication and
light sources, etc.
REFERENCES
Smart WH, Subramanian K., 2000. The use of silicon
microfabrication technology in painless blood glucose
monitoring. Diabetes Technol Ther.
Pfützner A, Caduff A, Larbig M, Schrepfer T, Forst T.,
2004. Impact of posture and fixation technique on
impedance spectroscopy used for continuous and non-
invasive glucose monitoring. Diabetes Technol Ther .
Rossetti P, Porecellati F, Fanelli CG, Bolli GB., 2006.
Evaluation of the accuracy of a microdialysis-based
glucose sensor during insulin-induced hypoglycaemia,
its recovery, and post-hypoglycaemic hyperglycaemia
in humans. Diabetes Technol Ther.
Cass AEG, Francis DG, Hill HAO, Aston WJ, Higgins IJ,
Plotkin EV., 1984. Ferrocene-mediated enzyme
electrode for the amperometric determination of
glucose. Anal Chem.
Shen YC, Davies AG, Linfield EH, Taday PF, Arnone
DD., 2003 The use of Fournier-transform infrared
spectroscopy for the quantitative determination of
glucose concentration in whole blood. Phys Med Biol.
Esenaliev RO, Larin KV, Larina IV., 2001 Non-invasive
monitoring of glucose concentration with optical
coherence tomography. Optics Letter.
Dieringer JA, McFarland AD, Shah NC, Stuart DA,
Whitney AV,Yonzon CR., 2006. Surface-enhanced
Raman spectroscopy: new materials, concepts,
characterization tools, and applications. Faraday
Discuss.
Cameron BD, Baba JS, Coté GL., 2001. Measurement of
the glucose transport time delay between the blood and
aqueous humour of the eye for the eventual
development of a non-invasive glucose sensor.
Diabetes Technol Ther .
Malchoff CD, Shoukri K, Landau JI, Buchert JM., 2002.
A novel non-invasive blood glucose monitor. Diabetes
Care.
Noro, M., Suzuki, K., Kishi, N., Hara, H.,Watanabe, T.,
Iwaoko, H., 2003. CO2/H2O gas sensor using tunable
Fabry-Pérot filter with wide wavelength range. Proc.
IEEE MEMS 2003 Conf.
Stupar, P. A., Borwick, R. L., DeNatale, J. F., Kobrin, P.
H., Gunning, W. J., 2009. MEMS tunable Fabry-Pérot
filters with thick, two sided optical coatings. Proc.
IEEE Transducers 2009 Conf.
N. Neumann, M. Ebermann, E. Gittler, M. Meinig, S.
Kurth, and K. Hiller., 2010. Uncooled IR sensors with
tunable MEMS Fabry-Pérot filters for the long-wave
infrared range. In Sensors, 2010 IEEE.
Macleod, H.A., 2001. Thin-Film optical filters, IoP,
Bristol and Philadelphia.
Tunable
FP filter
detector
Testing
circuit
Voltage
supply
PC
TunableFabry-PérotFilterforOpticalGlucoseMonitoring
175