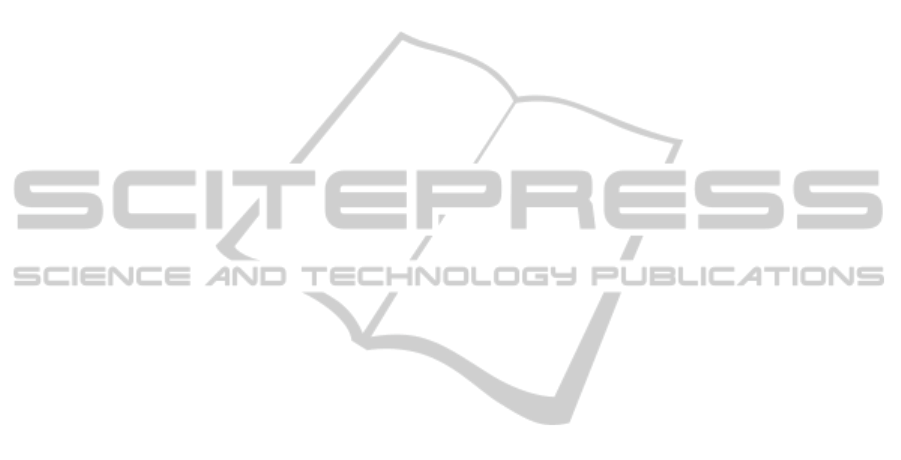
expansion of carbon dioxide gas in high pressure
chamber. The carbon dioxide has some solubility in
the PLGA. Thus, when the high pressure of carbon
dioxide is applied to the PLGA scaffold and then
released quickly, it becomes to expand in the PLGA
matrix. Resultantly, the pores are developed at the
places where the gas existed. However, the
disadvantage of this method is that it is also hard to
connect between the pores. Thus, the salt leaching
method is combined together to make porous
structure. However, there has been no reprot to
apply this method to make a porous bioactive
composite material, yet.
In this study, we prepare the PLGA/calcium
silicate composite by the solvent casting method and
then a gradient pore structure was introduced using
the expansion of carbon dioxide gas in a high
pressure chamber.
2 MATERIALS AND METHODS
2.1 Preparation of Calcium Silicate
Powders
The calcium silicate (SiO
2
-CaO) particles were
prepared with the starting composition of
70SiO
2
30CaO in molar ratio. The calcium silicate
particles were prepared by hydrolysis and
polycondensation of tetraethyl orthosilicate (TEOS,
Nacalai Tesque) in calcium nitrate tetrahydrate
(Nacalai Tesque) and polyethylene glycol (PEG,
Aldrich) in aqueous solution. The molecular weight
of PEG used in this experiment was about 10000.
PEG and calcium nitrate tetrahydrate were
dissolved in distilled water and then concentrated
nitric acid (60 wt%, Nacalai Tesque) was added.
TEOS was added to the above solution under
stirring. After 20 min, the solution was transferred to
a polystyrene box with its top sealed tightly, and
kept at 40C in a convection oven for gelation and
aging for 1 day. The obtained wet gel was immersed
in distilled water for 3 h with the distilled water
renewed every hour. After the wet gel was dried at
40C for 7 days, it was heated at 600C for 2 h and
then pulverized using a planetary ball mill.
2.2 Preparation of a PLGA/Calcium
Silicate Composite
The 90PLGA/10SiO
2
-CaO composite (in wt%) was
made by the solvent casting. PLGA powder
(90PLA10PGA, IV 0.55 ~ 0.75, Medisorb,
Alkemes) was dissolved in chloroform (4%) after
which SiO
2
-CaO particles were added to the
solution. After stirring vigorously for 1 h, the
mixture was poured into a Teflon mould and dried
under ambient conditions.
2.3 Preparation of a PLGA/Calcium
Silicate Composite with a Gradient
Pore Structure
The 90PLGA/10SiO
2
-CaO composite was loaded
into the high carbon dioxide pressure chamber and
then carbon dioxide gas was introduced into the
chamber achieving final pressure of 10 MPa. The
specimens were allowed to equilibrate and saturate
with the carbon dioxide gas for 3 days, forming a
single phase PLGA/CaO-SiO
2
/CO
2
gas solution at
room temperature. Subsequently, the carbon dioxide
gas was quickly released bringing the chamber to
ambient pressure. (Mooney et al., 1996)
2.4 Bioactivity Test
The bioactivity of the PLGA/CaO-SiO
2
composite
was assessed by evaluating its capability to form low
crystalline hydroxyl carbonate apatite on its surface
in simulated body fluid (SBF). (Kokubo et al., 1990)
The SBF was prepared by dissolving reagent grade
NaCl, NaHCO
3
, KCl, K
2
HPO
4
·3H
2
O, MgCl
2
·6H
2
O,
CaCl
2
, and Na
2
SO
4
in ion exchanged distilled water.
Their ionic concentrations were Na
+
142, K
+
5.0,
Mg
2+
1.5, Ca
2+
2.5, Cl
-
147.8, HCO
3
-
4.2, HPO
4
2-
1.0, SO
4
2-
0.5 (in mM). The solution was buffered at
pH 7.4 with tris(hydroxymethyl) aminomethane
((CH
2
OH)
3
CNH
2
) and 1 M hydrochloric acid (HCl)
at 36.5
o
C.
Specimen disks 12 mm in diameter by 4
mm in thickness were cut, sterilized under UV lamp
for 30 minutes, and then dried on a clean bench.
Subsequently, the specimens were incubated in 30
mL of the SBF at 36.5
o
C for 7 days. After
incubation, the PLGA/CaO-SiO
2
composite was
removed, gently rinsed with ion-exchanged distilled
water several times, and dried at room temperature.
2.5 Characterization
Microstructure was observed by a field emission
scanning electron microscopy (FE-SEM; S-4700,
Hitachi). The crystal phase of the specimens before
and after soaking in the SBF was evaluated by a thin
film X-ray diffractometry (TF-XRD; D8 Discover,
Bruker).
BIODEVICES2013-InternationalConferenceonBiomedicalElectronicsandDevices
240