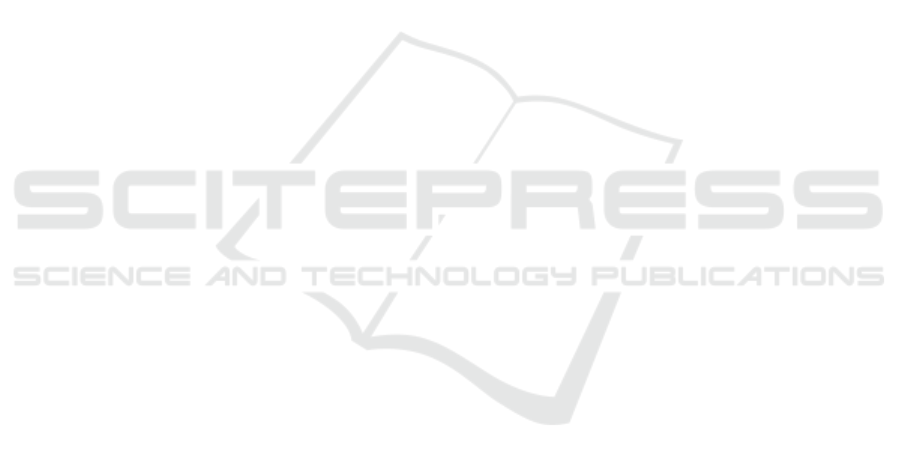
SIN
X
/SIO
2
STACKED SENSITIVE THIN FILM
FOR ISFET-BASED CHEMICAL AND BIOCHEMICAL SENSORS
Preparation and Characterization of the Stacked Thin Films and Sensors
J. F. Souza
1,2,3
, M. B. Lima
4
, I. Doi
1,2
, P. J. Tatsch
1,2
, J. A. Diniz
1,2
and J. L. Gonçalves
3
1
School of Electrical and Computer Engineering, University of Campinas, Av. Albert Einstein 400, Campinas, SP, Brazil
2
Center for Semiconductor Components, University of Campinas, R. João Pandiá Calógeras 90, Campinas, SP, Brazil
3
Center for Information Technology Renato Archer, Rod. D. Pedro I (SP – 65) Km 143, 6, Campinas, SP, Brazil
4
Superior School of Sciences of the Health, University of Amazonas, Av. Carvalho Leal, 1777, Manaus, AM, Brazil
Keywords: SiNx/SiO
2
, Silicon nitride thin film, ISFET, Chemical sensor, Biochemical sensor.
Abstract: In this work, nitrogen rich SiN
x
thin film was deposited on SiO
2
/p-Si (100) substrate by low pressure
chemical vapour deposition (LPCVD). The film was physically characterized using techniques such as
Fourier transform infrared spectroscopy (FTIR), atomic force microscopy (AFM) and ellipsometry. The
biocompatibility of such film was investigated by FTIR. Using a set of metal insulator semiconductor field
effect transistors (MISFETs) and ion sensitive field effect transistors (ISFETs) fabricated, electrical
characteristics and sensing properties were investigated. The biocompatibility of the SiN
x
film and the
electrical quality of the SiN
x
/SiO
2
/p-Si interface obtained suggests that SiN
x
/SiO
2
is an adequate insulator
on ISFET based chemical and biochemical sensors.
1 INTRODUCTION
LPCVD Si
3
N
4
films are used as the sensitive
material in miniaturized ISFET-based chemical and
biochemical sensors. Such devices have been used
for example for further surface modifications
allowing for antigen-antibody biosensor
applications. In vivo studies classify Si
3
N
4
as a
biocompatible material (Gustavsson et al., 2008).
The first ISFET was applied by Bergveld (Bergveld,
1970) to a biosensor for measuring ion concentration
in nerve tissues. The latest investigations related to
the ISFET-based biosensor are extended to
immunosensing (Schenck, 1978, Schöning and
Poghossian, 2002) and DNA hybridization sensing
(Souteyrand et al., 1997, Pouthas et al., 2004).
Ultrasensitive detection of biomolecules using
various types of one-dimensional nanostructures
such as carbon nanotubes (Villamizar et al., 2008),
graphenes (Cheng et al., 2010) and nanowires
(Knopfmacher et al., 2010) has attracted broad
research interest during the past decade due to their
high surface-to-volume ratio. An important
parameter, the slop of characteristic, is directly
related with sensitivity of the sensor. These aspects
have not been yet convincingly reported, for this
reason, this work reports the use of SiN
x
/SiO
2
stacked sensitive thin films that are biocompatible
and present high quality of the electrical interface,
increasing the sensitivity and making possible a
direct electrical detection of charged molecules.
2 EXPERIMENTS
2.1 SiN
x
/SiO
2
Stacked Sensitive Thin
Film Preparation
The (100)-orientated 1-10 Ω.cm p-type silicon
wafers were used as substrates. The 5 nm thick
thermally grown silicon oxide (SiO
2
) film was
prepared using a conventional furnace at 1000
o
C for
1 minute, in a high purity oxygen atmosphere. Then,
the SiN
x
layer, a sensing membrane, was deposited
by LPCVD in a SiCl
2
H
2
/NH
3
gas mixture
atmosphere at 740
o
C. The base and work pressure
of the chamber were 10 mTorr and 0.57 Torr,
respectively. The flow rate of SiCl
2
H
2
was fixed at
23 standard cubic centimeters per minute (sccm),
while the flow rate of NH
3
was 60 sccm. All
302
Souza J., Lima M., Doi I., Tatsch P., Diniz J. and Gonçalves J..
SINX/SIO2 STACKED SENSITIVE THIN FILM FOR ISFET-BASED CHEMICAL AND BIOCHEMICAL SENSORS - Preparation and Characterization of
the Stacked Thin Films and Sensors.
DOI: 10.5220/0003721603020306
In Proceedings of the International Conference on Biomedical Electronics and Devices (BIODEVICES-2012), pages 302-306
ISBN: 978-989-8425-91-1
Copyright
c
2012 SCITEPRESS (Science and Technology Publications, Lda.)