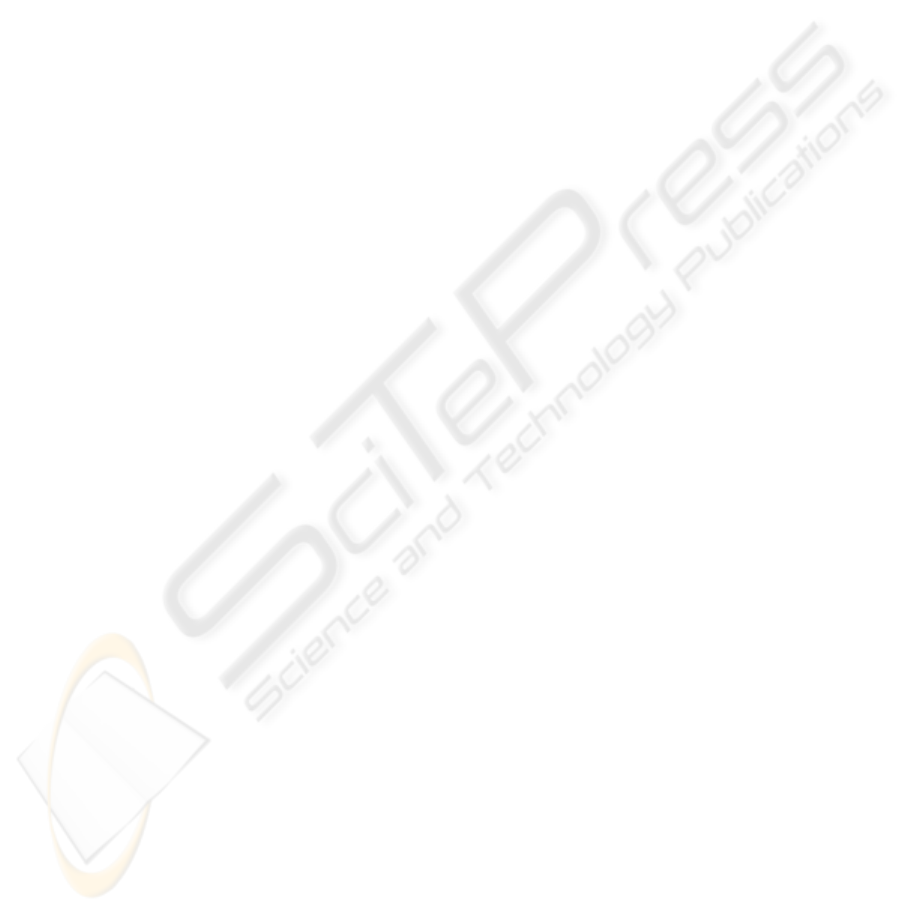
Multiple applicators (arrays) with integrated
temperature sensors could be used in order to treat
larger tissue volumes and more accurately estimate
the temperature distribution through the combined
application of bio-heat equation and tomography
algorithms.
The pliability of the miniaturized coaxial cable
and of the associated silicon catheter make easier the
insertion of the applicator using the natural way of
the body or minimally invasive surgical procedures.
Many coaxial applicators for hyperthermic
treatments show a heating pattern characterized by a
typical tear drop shape. The implementation of a
coaxial choke in the MHA investigated in this paper
reduces appreciably the drop tail and allows to
precisely localize the tissue volume involved during
the microwave treatment. Moreover the high degree
of miniaturization due to the availability of
miniaturized coaxial cable to use in medium-high
power applications (dimensions about 1-2 mm in
diameter are easily available), permits to extend the
clinical applications of this minimally invasive
applicator. Hyperthermic treatments of bile-ducts in
cancer therapy, impracticable in the past for the
restrict dimensions of the ducts, could be possible
nowadays as well other delicate surgical
interventions that require high precision and reduced
invasivity.
The originality of this MHA, from an
engineering point of view, lies in the peculiar
integration of the metallic wires of a low cost
temperature sensor inside of the choke body without
perturbing the SAR distribution.
The invasivity of the clinical hyperthermic or
thermo-ablation treatment is highly reduced using
this type of applicator because in fact no separated
insertions for temperature probes, no additional
external electrodes as for RF treatments or any other
kind of devices are required. Therefore many deep-
seated tumors (e.g. certain brain, liver,
gastrointestinal or gynaecological tumours), could
be effectively and easily treated with the proposed
MHA.
The integrated temperature sensing element
permits to accurately monitor the maximum
temperature reached into the tissue and it can be
used to close the control loop of a specific
microwave hyperthermic or ablative process by
defining the appropriate thermal-dose to be
administered to the lesion.
By monitoring the temperature in time domain,
very useful data on blood perfusion rate, thermal
conductivity and specific heat could be directly
extrapolated by the bio-heat transfer equation and
used to construct more complex and realistic
biological tissue models. As well thermal properties
difference between healthy and pathological tissue
could be relieved in order to extrapolate diagnostic
information.
REFERENCES
Turner, F., 1986. Interstitial equal-phased arrays for EM
hyperthermia. IEEE Trans. Microwave Theory Tech.,
vol. 34, no. 5, pp. 572-578.
Tumeh, A.M., Iskander, M.F., 1989. Performance
comparison of available interstitial antennas for
microwave hyperthermia. IEEE Trans. Microwave
Theory Tech., vol. 37, no. 7, pp. 1126-1133.
Camart, J.C., Despretz, D., Chive, M., Pribetich, J., 1996.
Modeling of various kinds of applicators used for
microwave hyperthermia based on the FDTD method.
IEEE Trans. Microwave Theory Tech., vol. 44, no. 10,
pp. 1811-1818.
Lin, J.C., Wang, Y. 1987. Intertitial microwave antennas
for thermal therapy. Int. J. Hyperthermia. vol. 3, no. 1,
pp. 37-47.
Cerri, G., De Leo, R., Primiani, V.M. 1993. Thermic
endfire’ interstitial applicator for microwave
hyperthermia. IEEE Trans. Microwave Theory Tech.,
vol. 41, no. 6, pp. 1135-1142.
Saito, K., Hayashi, Y., Yoshimura, H., Ito, K., 2000.
Heating characteristics of array applicator composed
of two coaxial-slot antennas for microwave
coagulation therapy. IEEE Trans. on Microwave
Theory and Techniques, vol. 48, no. 11, pp. 1800-
1806.
Bowman, R.R. 1976. A probe for measuring temperature
in radio-frequency-heated material. IEEE Trans. on
Microwave Theory and Techniques, pp. 43-45.
Longo, I., Biffi Gentili, G., Cerretelli, M., Tosoratti, N.,
2003. A Coaxial Antenna with Miniaturized Choke for
minimally Invasive Interstitial Heating. IEEE Trans.
on Biomedical Engineering, vol. 50, no. 1, pp. 82-88.
Jones, K., Mechling, J.A., Trembley, B.S. 1988. SAR
distributions for 915 MHz interstitial microwave
antennas used in hyperthermia for cancer therapy.
IEEE Trans. on Biomedical Engineering, vol. 35, no.
10, pp. 851-857.
Biffi Gentili, G., Leoncini, M., Trembly, B.S., Schweizer
S.E. 1995. FDTD Electromagnetic and Thermal
Analysis of Intertitial Hyperthermic Applicators. IEEE
Trans. on Biomedical Engineering, vol. 42, no. 10, pp.
973-980.
CST Studio Suite 2006, Computer Simulation Technology
GmbH, D-64289 Darmstadt, Germany.
Berenger, P. 1994. A perfectly matched layer for the
absorption of electromagnetic waves. J. Computat.
Phys. vol. 114, no. 2, pp. 185-200.
Pennes, H.H. 1948. Analysis of tissue and arterial blood
temperatures in the resting human forearm. J. Appl.
Physiol. vol. 85, no. 1, pp. 93-122.
BIODEVICES 2008 - International Conference on Biomedical Electronics and Devices
118