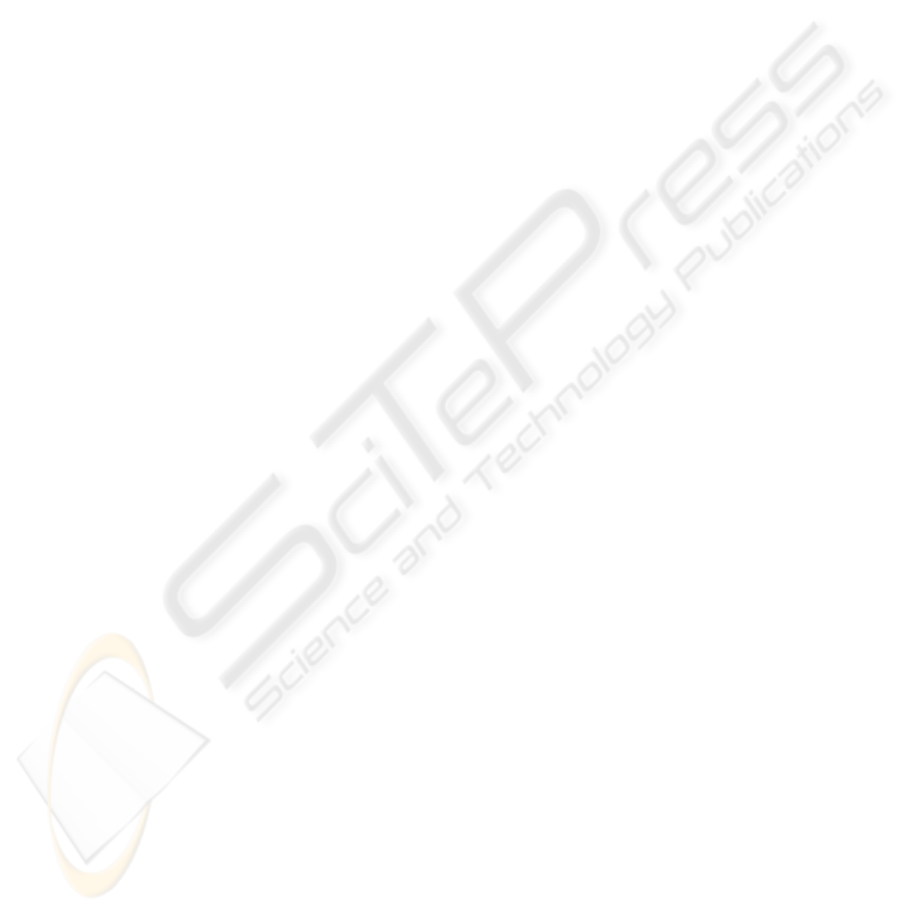
simulate a worst case situation, the sites of both
operators are not co-located and the interoperator
spatial offset is equal to the cell radius (Hiltunen,
2002).
It was found that the inter-frequency
interference impact on the capacity was minimal,
when compared with the intra-frequency
interference. The reason for this result lies in the
fact that there are too many BSs from the same
carrier interfering with each other.
The next step taken was to identify scenarios
where the ACI played a significant role, at least as
important as the interference coming from the
connections working on the same carrier. Following
simple scenarios, where just a few BSs and two
carriers were taken into account, the analysis
developed to encompass broader environments
simulating urban centres with many antenna sites
and three carriers.
A simple map was used as an entrance
parameter to the simulator, with no additional
information, apart from UE and BS positions. When
placing the BSs of two different carriers, one must
decide whether they are co-located, i.e. both cells
lie on the same site, or not. In the latter situation, it
is assumed that the worst case for ACI happens, i.e.
the adjacent channel site is located at the coverage
edge of the first channel cell.
The simulator used to achieve this analysis was
static, using a Monte-Carlo evaluation method. As a
result, the users were placed randomly on the map.
Following the iterative process, only the
connections with sufficient Eb/No (or signal to
noise ratio - SNR) for the appointed service were
considered to be served by the system. This
simulator was adapted from the previous one
described in (Laiho et al., 2002) and (Wacker et al.,
2001). By examining many static situations,
referred to as snapshots, network capacity is
estimated through the average number of the served
users (Povey et al., 2003).
The bit rates tested in this study were chosen in
accordance with the services expected to be offered
by operators in the first implementation phase. In
this case, 12.2 kbps with CS (Circuit Switching), 64
kbps with CS and, finally, 128 kbps in downlink
and 64 kbps in uplink using PS (Packet Switching).
The results are presented taking into account users
accessing one of these three types of services.
The UE power classes considered for
determining the maximum output power were class
3 (24 dBm) for voice and class 4 (21 dBm) for data
services (3GPP 25.101). The BS maximum output
power used was 43 dBm.
Two types of antennas were chosen to simulate
macro and micro BS: for the macro BS, tri-
sectorized antennas with 18 dBi of gain, and for the
micro BS, omni-directional antennas with 4 dBi of
gain.
Two different propagation models were
considered to calculate the path loss according to
the characteristics of the environment (both for
outdoor propagation). For rural scenarios the COST
231 Hata model was used. The main input
parameters for the model are the UE antenna
heights, 1.5 m, and BS antenna heights, 35 m. For
the urban environment the propagation model
applied was COST 231 Walfish-Ikegami. The main
parameters used are UE antenna heights, 1.5 m, BS
antenna heights, between 10 and 25 m (depending if
they are macro or micro), street width, 20 m,
building separation, 40 m, and building height, 12
m.
4 RESULTS
In the extended study that originated this paper, a
wide range of scenarios and environments were
considered (Figueiredo and Matos, 2003). Urban,
rural and motorway environment were tested using
layers containing twenty-three macro cells placed in
the form of a grid. Furthermore, eight scenarios
with only a few antennas (five at the most) were run
to simulate specific situations using macro and
micro cells. The dense urban environment was
simulated, by using macro cells layers and micro
cells to cover identified hotspots. In this paper, only
the three most significant tests will be presented.
At the end of each simulation, the outputs were
analysed. Apart from the maps indicating the BS
and UE position, the network’s capacity (measured
in average number of served users) and the capacity
loss (when compared with no ACI), parameters like
the ratio between sources of interference were also
analysed in (Figueiredo and Matos, 2003). The
interference sources considered in the results
included the interference coming from the adjacent
channel, the interference from the same channel
from neighbouring cells and the interference from
the same cell (due to the other users connected to
the same BS).
A maximum load of 50 % was allowed in the
radio interface.
4.1 Case 1: Small scale networks with
two operators
This scenario was developed to study the impact of
a new micro BS placed by an operator to cover a
hotspot in the middle of an existing network of
macro BSs from the adjacent carrier competitor.
ICETE 2004 - WIRELESS COMMUNICATION SYSTEMS AND NETWORKS
76